By Kim Burnett and Christopher Wada
Results from a recent statistical exercise suggest that by the end of the 21st century, Hawaii will likely see a 5-10% reduction in precipitation during the wet season and a 5% increase during the dry season (Timm and Diaz 2009). Given that approximately 70% of normal precipitation falls during the wet season, Hawaii is facing an overall decline in annual precipitation, and thus a decline in groundwater-recharge (how much water goes toward refilling our critical aquifers*). Meanwhile, water tables and streamflow have already been declining as a result of both increased groundwater withdrawals and the warming climate (Bassiouni and Oki 2012).
Drawdown of existing groundwater stocks is likely still decades away, meaning there’s still time to do something about it. One option is watershed conservation. Watershed conservation, of course, has costs and those costs can appear quite high in the near-term. For example, the construction of pig fencing — one tactic for achieving watershed conservation — can cost between $92,000 and $159,000 per mile, not including helicopter time and materials. Conservation activities, however, can generate much larger benefits over the long run. Estimating these benefits is the topic of our new working paper: Optimal groundwater management when recharge is declining: A method for valuing the recharge benefits of watershed conservation.
To arrive at these estimates, streamflow and evapotranspiration projections (Safeeq and Fares 2012) and rainfall projections (Timm Diaz 2010) were used to construct two potential climate change scenarios:
(i) a precipitation decline of 5.3% and subsequent recharge decline of 8.5% by 2100 (baseline) and
(ii) a precipitation decline of 1.9% and a subsequent recharge decline of 3.7% by 2100 (conservative)
Using a dynamic economic-hydrologic optimization model (read the working paper here for more on the model) and based on these scenarios, we looked at the Pearl Harbor aquifer and calculated a net present value (NPV) based on the stream of future benefits (expressed in dollars) derived from having this water resource available at current recharge rates. We then calculated NPV for the aquifer in each of the two climate change scenarios. The amount of value “lost” (the difference between the NPV at current recharge rates and the NPV at lower recharge rates) represents the potential benefit of conservation.
The net present value (NPV) of the Pearl Harbor aquifer is approximately $7.886 billion at the current rate of natural recharge. Our two climate change scenarios reduce the value:
(i) If a decline in precipitation reduces recharge by 3.7% the value drops to $7.722 billion. (potential benefit of $163.9 million)
(ii) If recharge is reduced by 8.5% the value drops even further to $7.538 billion. (potential benefit of $347.7 million)
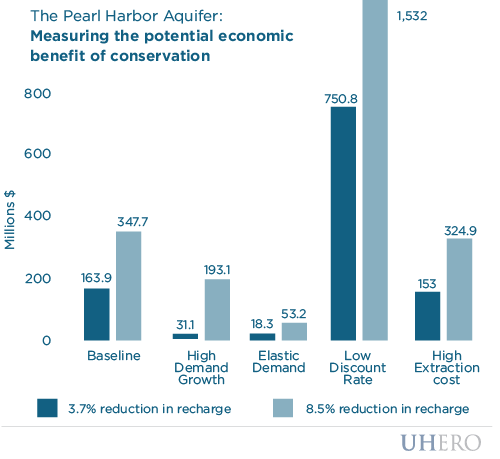
We conducted a sensitivity analysis on several of the model’s parameters (see results in figure above and explanation of parameters below). We found that maintaining the current level of recharge in the aquifer represented a value of anywhere between $31.1 million and $1.5 billion. In addition to increasing welfare by lowering the scarcity value of water in both the near term and the future, enhancing recharge delays the need for costly alternatives like desalination.
The enormous dollar values in the Pearl Harbor aquifer example illustrate the kinds of huge benefits conservation can generate. Pig fencing may cost a lot, but it still pales in comparison to the potential tens of millions – or even billions – that can be had from such conservation projects over the long term. When other ecological services provided by forested watersheds are considered (e.g. those related to species habitat, subsistence, hunting, aesthetic value, commercial harvest, protection against flooding and sedimentation, and ecotourism), the value of watershed conservation may be much higher.
About the Chart: Valuing the Conservation of the Pearl Harbor Aquifer
1. Our baseline assumed demand for water would grow by 1% each year (based on historical data for population and per capita income growth).
2. The high growth scenario assumes demand for water grows at triple that rate (3% each year).
3. Many past studies suggest that demand for water is fairly constant given changes in price, i.e. demand is “inelastic”. However, recent research suggests that consumers facing increasing block prices (the pricing structure currently used in Hawaii) may be more responsive to price changes. The high elasticity scenario assumes that the price elasticity** of demand is -0.5, twice the value of the baseline scenario.
4. The discount rate adjusts the value of future benefits or costs to reflect the desire to accrue benefits sooner and costs later. In the low discount rate scenario, the discount rate is one third of its baseline value (0.01), which means that weighting of net benefits accrued to current and future consumers is closer to being equal.
5. Because the price derived from the optimization model used reflects both the extraction cost and the scarcity value of water, with the scarcity value of water being relatively large, the high extraction cost scenario (where the cost coefficient is double its baseline value) does not substantially change the NPV.
*Hawaii’s groundwater provides nearly 99% of Hawaii’s domestic water and roughly 50% of all freshwater used throughout the state (Gingerich and Oki 2000).
**Price Elasticity: The price elasticity of demand measures the percentage change in quantity demanded resulting from a one percent change in price. In other words, it is a measure of consumer responsiveness to price changes. Demand for a good is said to be inelastic when elasticity is less than one and elastic when elasticity is greater than one. In our baseline scenario, for example, the -0.25 value for demand elasticity means that a user consuming 10,000 gallons of water per month at a price of $5 per thousand gallons would reduce consumption by 25 gallons per month if the price of water is increased to $5.05 per thousand gallons.
References
Bassiouni M, Oki DS (2012) Trends and shifts in streamflow in Hawai‘i, 1913–2008. Hydrol Process. doi:10.1002/hyp.9298 Gingerich SB, Oki DS (2000) Ground water in Hawaii, U.S. Geological Survey Fact Sheet 126-00
Safeeq M, Fares A (2012) Hydrologic response of a Hawaiian watershed to future climate change scenarios. Hydrol Process 26:2745–2764
Timm O, Diaz, HF (2009) Synoptic-Statistical Approach to Regional Downscaling of IPCC Twenty-First-Century Climate Projections: Seasonal Rainfall over the Hawaiian Islands. J Climate 22:4261-4280.
This research is forthcoming in the peer-reviewed journal, Environmental Economics and Policy Studies (Burnett and Wada 2014). For more applications of economic principles to natural resource and environmental management problems, visit UHERO’s Project Environment.
BLOG POSTS ARE PRELIMINARY MATERIALS CIRCULATED TO STIMULATE DISCUSSION AND CRITICAL COMMENT. THE VIEWS EXPRESSED ARE THOSE OF THE INDIVIDUAL AUTHORS. WHILE BLOG POSTS BENEFIT FROM ACTIVE UHERO DISCUSSION, THEY HAVE NOT UNDERGONE FORMAL ACADEMIC PEER REVIEW.